Many of us have asked the question as children: why is the sky blue? Some believe that its color is influenced by the reflection of sunlight from the ocean. Others are convinced it’s due to water vapor in the atmosphere. So why is our sky is painted blue? Let’s explore this and find out if there are planets where the skies are colored some other way.
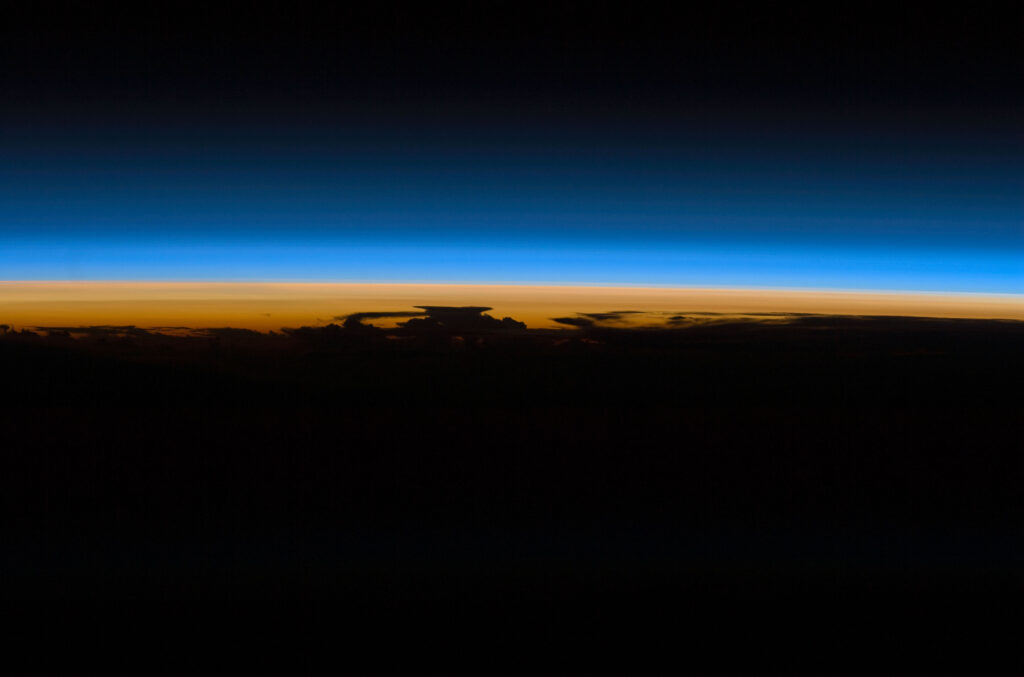
Our life experience tells us that the color of the sky is somehow related to sunlight: it’s blue when the Sun is above the horizon, but after it sets, it darkens and eventually becomes black with a sprinkling of stars. This is what the sky looks like on planets and their moons that lack an atmosphere.
Now, let’s take a closer look at the Sun as it descends to the horizon. It’s easy to notice that the lower it gets, the redder its color becomes. Sometimes it turns completely red and dim enough to be safely observed with the naked eye. Furthermore, the Moon near the horizon also appears noticeably “redder.” Why does this happen, and how is it related to the color of the sky?
In reality, both of these phenomena are caused by the same physical process known as molecular scattering of light. Let’s delve into it in more detail.
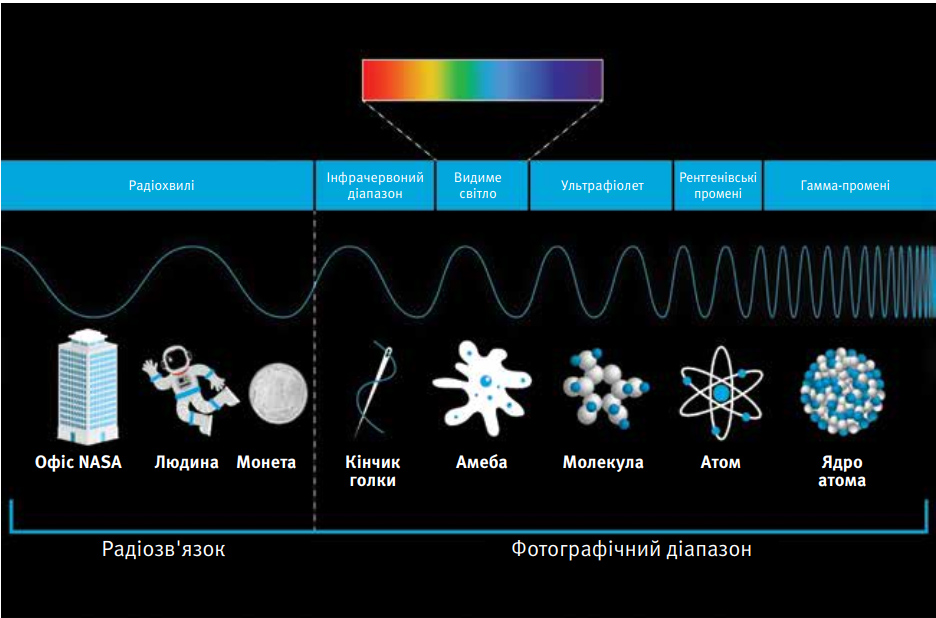
The Sun emits energy in the form of photons (quantums of light) with varying energy levels, corresponding to different wavelengths of radiation. The higher the energy, the shorter the wavelength. In the part of the spectrum to which the human eye is sensitive, we perceive these “differently energetic” photons as different colors of the rainbow. Short-wavelength radiation is seen as violet and blue, while long-wavelength radiation is seen as red and orange. Our eyes are most sensitive to yellow-green light with a wavelength of 555 nm. When photons with various wavelengths, roughly equal in intensity, reach our retina, our brain “synthesizes” them into white light.
In the absence of obstacles, photons travel in a straight line (for simplicity, we won’t delve into relativistic effects here) and reach us only from the “direct” source of light. This is why, for example, on the Moon, we see the Sun against a black sky. In addition to the Sun, we can only observe a few of the brightest stars and planets because the Sun’s glare causes our pupils to constrict, limiting our visual sensitivity. A full-fledged starry sky in space can be seen only by hiding from the blinding rays of our star and allowing our eyes some time to adjust to the darkness.
When an electromagnetic wave encounters an object significantly larger than its wavelength, it will be either reflected or absorbed by the object. However, when it interacts with something smaller than itself, it will change direction, sometimes quite significantly, up to nearly opposite (again, we won’t complicate things with electron interactions here). If there are enough of these “scattering particles” around us that are illuminated by the Sun, each of them will occasionally send a photon of light in our direction.
It’s easy to understand that in the case of Earth, these particles are atoms and molecules of atmospheric gases. They are the ones responsible for the illumination of the sky, not only during the day but also after the Sun has set, when its light is scattered by the upper layers of the atmosphere that it hasn’t yet disappeared behind (this is what creates twilight). But why does this illumination result in a blue sky?
Light scattering without changing the wavelength was studied in the late 19th century by the British physicist Lord Rayleigh, so this phenomenon became known as Rayleigh scattering. Among other things, the scientist found that such scattering occurs more actively as the wavelength of electromagnetic radiation closes to the size of the obstacle. In the visible spectrum, transitioning from the red end to the violet, this wavelength gradually approaches the size of the particles primarily making up the atmosphere: nitrogen and oxygen molecules. Consequently, they more effectively scatter the “cooler” colors (blue, cyan, violet). As a result, we see the sky as blue.
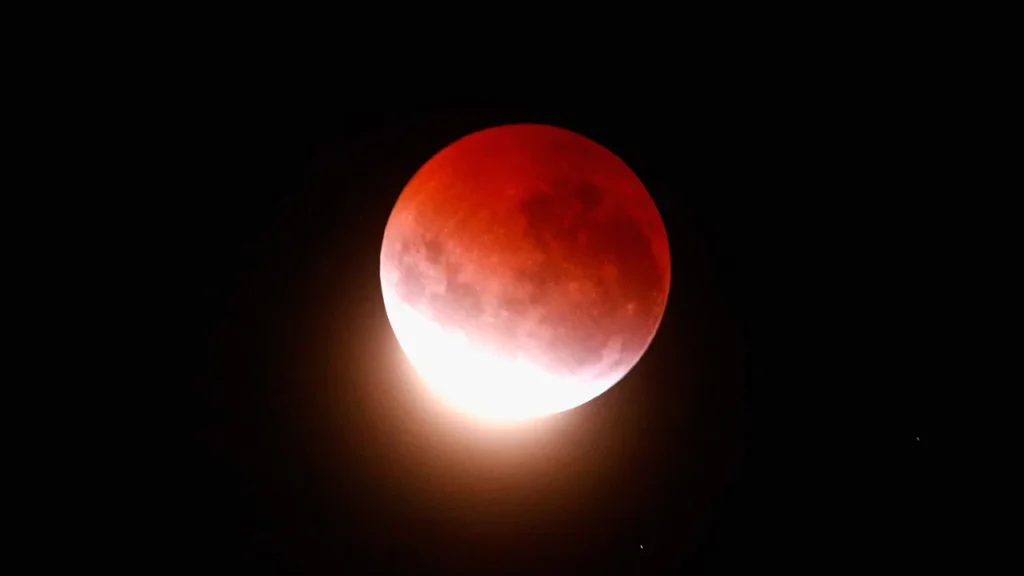
On the other hand, sunlight passing through the Earth’s atmosphere “loses” some of its short-wavelength photons, which is why the Sun appears somewhat yellowish when observed from the Earth’s surface. The closer it gets to the horizon, the thicker is the layer of atmospheric gases its rays have to pass through, and the fewer “blue” and “violet” colors they retain. This is why the Sun appears orange during sunrise and sunset. When volcanic eruptions or sandstorms release a lot of dust particles into the air, which are significantly larger than molecules, they actively scatter green and yellow rays as well. As a result, the Sun near the horizon takes on a blood-red color. It often appears this way in winter when the atmosphere becomes saturated with tiny ice crystal. All these effects can also be observed concerning the Moon.
Most commercial airliners fly at altitudes of 10.5 kilometers, where the light-blue sky gets much darker. For pilots of high-altitude military aircraft that climb above 16 kilometers, the sky becomes nearly black because 99% of the Earth’s atmospheric mass is concentrated below this altitude. This can be easily noticed during live broadcasts from video cameras mounted on the outer surface of rockets carrying payloads into space.
When the Moon passes through the Earth’s shadow during a total lunar eclipse, it almost never disappears completely. We can still see it, only tinted in an unusual brown color. This is due to the “mix” of solar rays that have passed through our planet’s atmosphere and, thanks to the refraction effect, have been “directed” toward the Earth’s shadow. Again, in this “filtered” light, there are almost no short-wavelength components — violet, blue, and green. As a result, a “bloody” color is formed, which in ancient times made representatives of many cultures consider such phenomena as harbingers of misfortune and catastrophes.
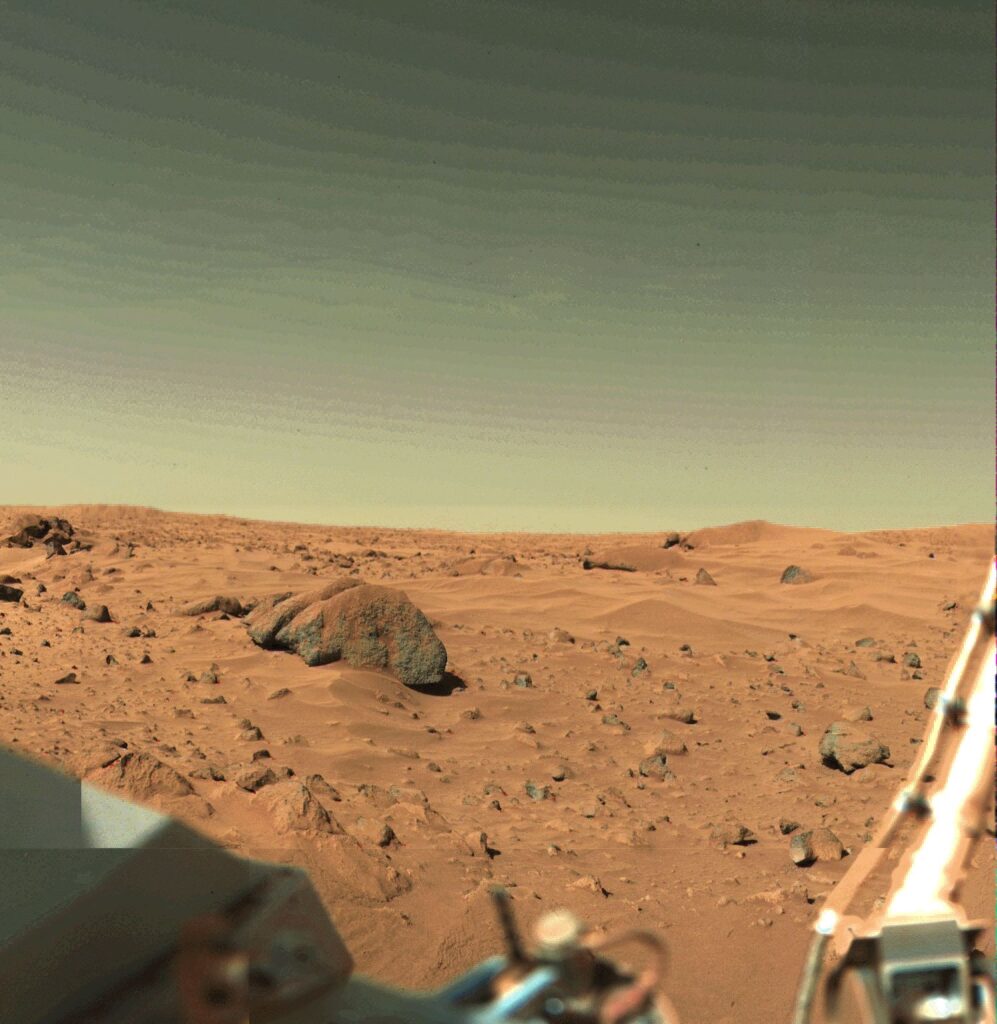
From everything that has been said, we can try to “calculate” what the color of the sky would be, for example, on Mars. The molecules of carbon dioxide, which make up almost 97% of its atmosphere, are about one and a half times larger than the molecules of oxygen and nitrogen, which primarily constitute Earth’s atmosphere. Therefore, the Martian sky would also be… blue, albeit with a slightly greenish tint. This is what we see in the photos transmitted from the surface of the Red Planet after color balancing to match human eye sensitivity. Though, it gets yellowish during mighty dust storms. It’s just dimmer than Earth’s sky and fades much faster after sunset: there are no lavish terrestrial twilight on Mars. As for future mountaineers who will climb Martian mountains that are at least 9-10 kilometers high, they will see absolutely “space-like” black skies above them.
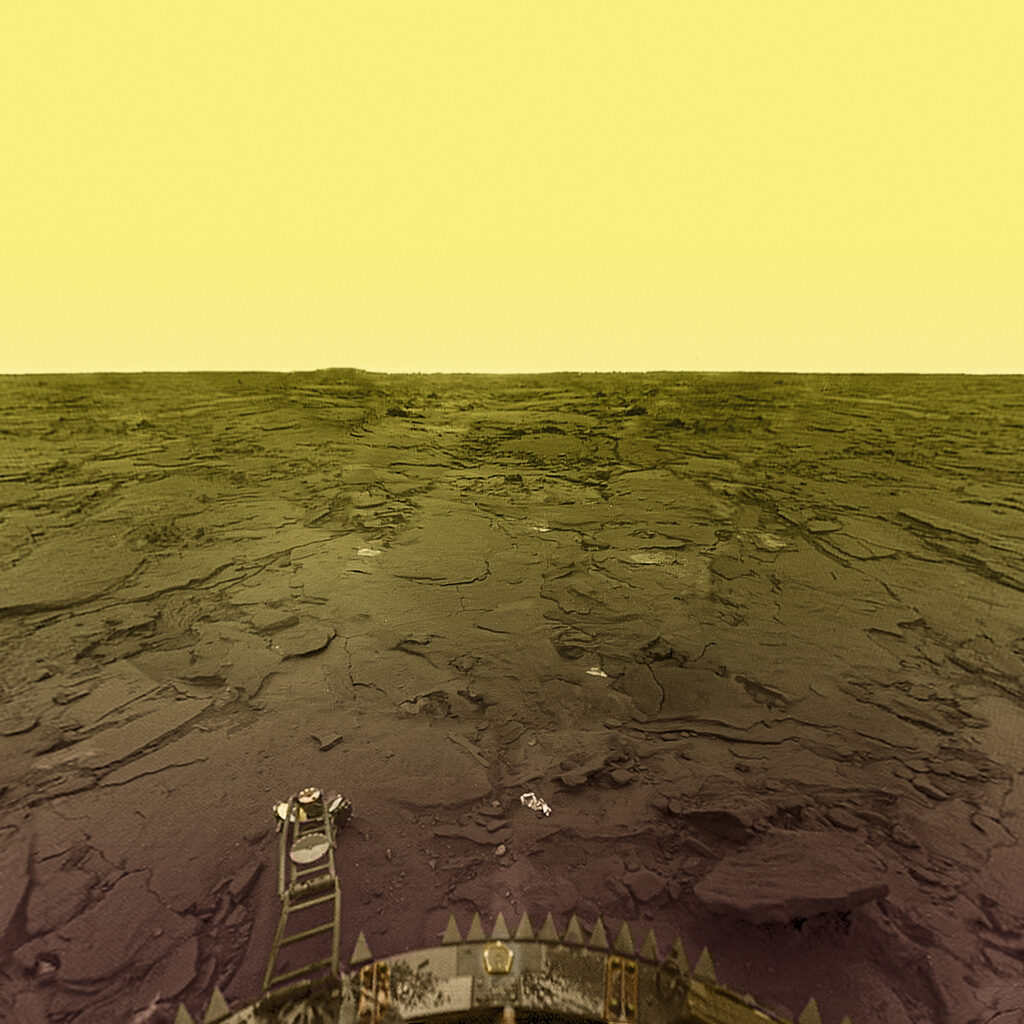
The sky will be blue on Jupiter, Saturn, Uranus, and Neptune because their atmospheres are primarily composed of hydrogen, which has the smallest molecules of all and therefore most effectively scatters short-wavelength end of the spectrum radiation. However, Venus and Saturn’s moon Titan have dense gaseous envelopes, so they can boast yellow and even orange skies. Here, the main role in scattering is played by atmospheric aerosols with sufficiently large particles: they interact actively not only with short-wavelength photons but even with “carriers” of red colors. Therefore, for most of the day, the Venusian sky would look yellow for a human eye (which was confirmed by color photos transmitted by the Soviet probes Venus-13 and Venus-14), and the dawns and evening twilights on Venus most likely would indeed be blood-red.