The near-Earth orbits into which spacecraft are launched are usually divided into the following categories. Low Earth orbits (LEO) are located at an altitude of 160 to 2000 km above the surface of our planet (in the first case, the orbital period is approximately 88 minutes, in the second — 127 minutes). Objects moving at altitudes of less than 200 km experience noticeable braking in the uppermost layers of the atmosphere and fall to the Earth fairly quickly. Therefore, orbits below 300 km are usually not used for satellites — their lifetime at such altitudes is relatively short. The upper value is determined by the inner boundary of radiation belts with an increased concentration of charged particles that can damage electronic equipment and cause serious damage to the health of astronauts.
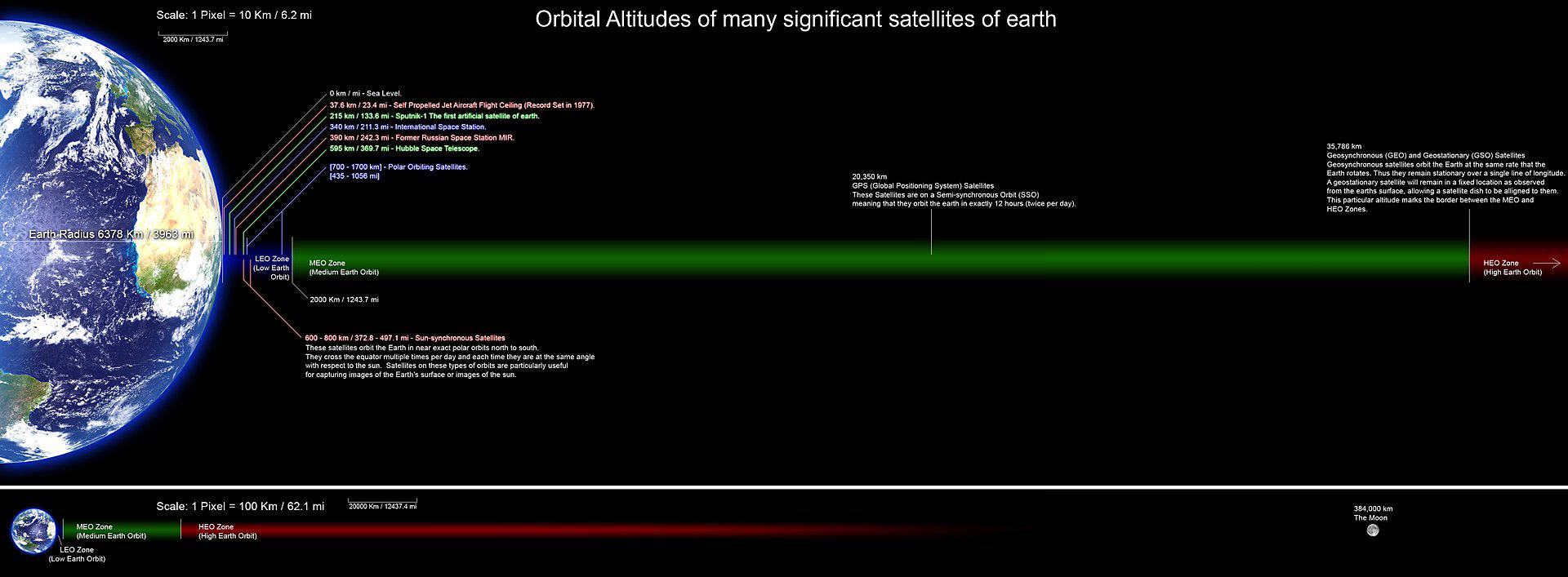
All manned space flights — with the exception of nine expeditions to the Moon as part of the American Apollo program — took place in the LEO region or were suborbital. The highest altitude (not counting lunar missions) was reached in September 1966 by the crew of the Gemini 11 spacecraft, which had an apogee of 1,374 km. At the moment, all inhabited orbital stations and the vast majority of other artificial Earth satellites are in low orbits. Most of the space debris is also concentrated on them.
The tangential velocity of the object (perpendicular to the direction to the center of the Earth) required to be at a stable LEO is approximately 7.8 km/s, decreasing with increasing altitude. To reach such orbits at launch from the Earth’s surface, a launch vehicle with a characteristic speed of 9.4 km/s is required — an additional 1.5—1.6 km/s is “spent” on aerodynamic and gravitational losses.
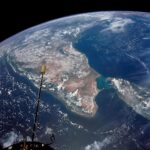
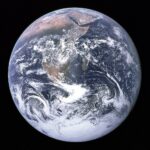
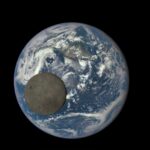
Many Earth remote sensing (ERS) satellites and military intelligence devices are brought to the LEO in order to survey ground objects from as close a distance as possible and achieve the highest possible resolution. The same orbits are occupied by some telecommunications satellites, since at this altitude they require less powerful signal amplifiers. However, each such spacecraft moves fast enough and covers a limited area of the earth’s surface, so in this case entire networks (“constellations”) are created from a variety of satellites — for example, there are more than 70 of them in the Iridium satellite telephone system.
A commonly used type of LEO — solar synchronous orbit (SSO), sometimes referred to as heliosynchronous — is calculated in such a way that an object located on it passes over any point of the earth’s surface at approximately the same local solar time. Usually such orbits have a height of about 800 km and an inclination of about 90° (their planes are almost perpendicular to the plane of the earth’s equator). If the satellite on the SSO is photographing the surface, the angle of incidence of the sun’s rays will be approximately the same on all its passes. For example, the LandSat-7 satellite can cross the equator 15 times a day, each time at 10:00 a.m. local time. For spacecraft that observe the Sun or require stable power supply through the use of photovoltaic panels, it is possible to select orbital parameters at which they will practically not fall into the shadow of the Earth. The orbits are chosen in such a way that the solar and lunar gravity causes their precession in an easterly direction by 360° per year (slightly less than 1° per day), compensating for the orbit of our planet around the Sun.
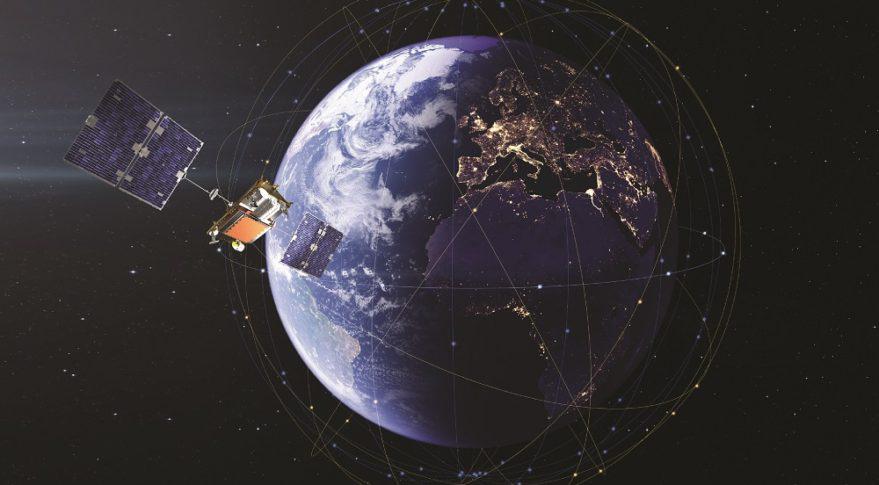
After the end of the functioning of artificial space objects, they are taken into a burial orbit, usually lying above their working orbit (in order to further weaken the influence of the atmosphere). In particular, low-orbit reconnaissance satellites with a nuclear power plant — including radar — are sent to an altitude of about 650-1000 km, where their estimated lifetime is about 2 thousand years. Often it is not the satellite itself that is sent there, but only the reactor nucleus. It is believed that during this period the most harmful radioactive isotopes will decay in it… or humanity will invent a way to dispose of dangerous equipment.
Above 2000 km there is a zone of so-called medium Earth orbits. They are used by a relatively small number of spacecraft — mainly research and navigation (in particular, GPS satellites move in orbits with a height of 20,350 km with an orbital period of 12 hours). The main problem in this area of space is related to radiation belts and the high-energy charged particles contained in them.
The upper boundary of the “middle” zone is marked by geosynchronous orbits (GSO) — they have a radius of 42,164 km, which corresponds to an altitude above sea level of 35,786 km. The period of orbits of objects in such orbits is equal to sidereal days (23 hours 56 minutes 4.1 seconds). Their special case is a geostationary orbit — circular and lying in the plane of the earth’s equator (0° latitude). A satellite moving along it actually turns out to be “hanging” over the same point of the Earth. Therefore, the receiving antenna, once directed at it, will not require further pointing. Obviously, such orbits are especially convenient for telecommunication devices, as well as specialized meteorological observatories monitoring a certain region.
If the orbit is inclined to the equator and has a small eccentricity, then when observed from Earth, the satellite will describe the “eight” in the sky during the day. In some cases, the “eight” can degenerate into an ellipse (as in the Canyon series satellites), and with significant eccentricity and zero inclination — into a straight line segment lying in the equatorial plane.
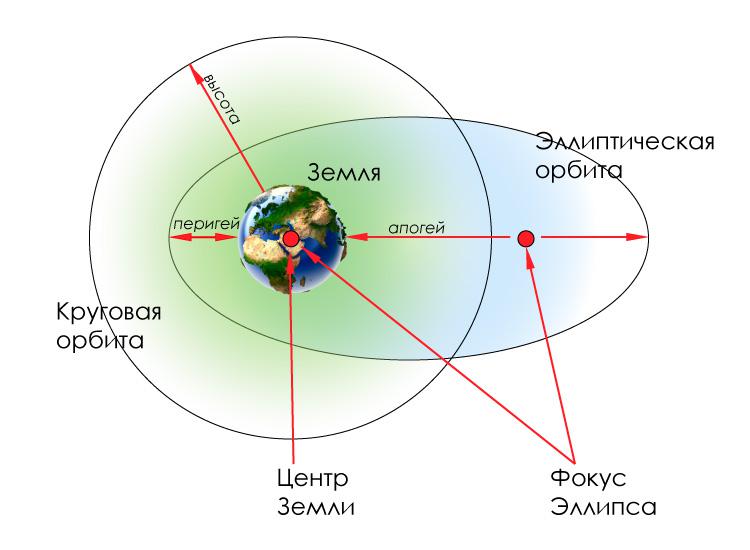
The ideal GSO is practically unattainable, since the spacecraft on it also experience attraction from the Moon and the Sun, the impact of the Earth’s magnetic field, solar wind and other extraneous disturbances that “collide” them from the point of standing. Therefore, a corrective propulsion system with a fuel reserve is provided on board geostationary satellites. In addition, such satellites are not visible from areas in the vicinity of the poles, extending to approximately 81° north and south latitude.
Twice a year (near the spring and autumn equinoxes) there are situations when telecommunication devices on the GSO are projected onto the solar disk. At this time, communication through them is difficult, and sometimes impossible at all.
The geostationary burial orbit is located about 200 km above the “standard” GSO. Satellites that have exhausted their resources or exhausted fuel reserves for onboard engines are sent there. Further, to a distance of about 300 thousand km (more precisely, to the Lagrange point L1 of the Earth-Moon system), there is a region of high near-Earth orbits. So far, they are used quite rarely — in particular, the TESS (Transiting Exoplanet Survey Satellite) space telescope is currently operating in this area of space.
Follow us on Twitter to get the most interesting space news in time
https://twitter.com/ust_magazine