The most common chemical element in the universe is hydrogen. It is found there both in atomic form and in the form of diatomic molecules (H2). Estimates of its concentration within the Milky Way give a value of 739 thousand atoms per million, that is, almost 74%. Stars and giant planets are mainly composed of hydrogen.
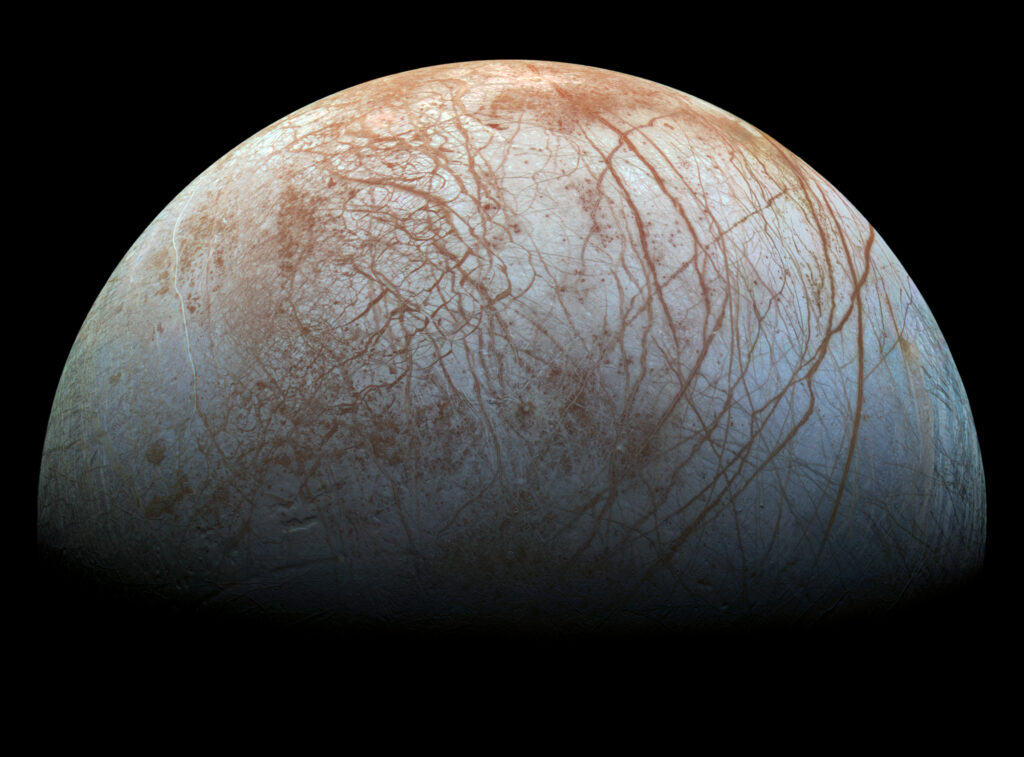
The next most common element is helium. It is partly formed during so-called primary nucleosynthesis shortly after the Big Bang, and partly is the product of thermonuclear reactions in the bowels of stars that provide them with the energy to glow. It can be said to be the noblest of the inert gases: it undergoes chemical reactions with other elements under very harsh conditions, and the resulting compositions are extremely unstable. So, if hydrogen in this universe “wishes” to combine with something, it will most likely do so with the third most common element, i.e. oxygen (it is almost a hundred times less abundant in our Galaxy than hydrogen and helium combined).
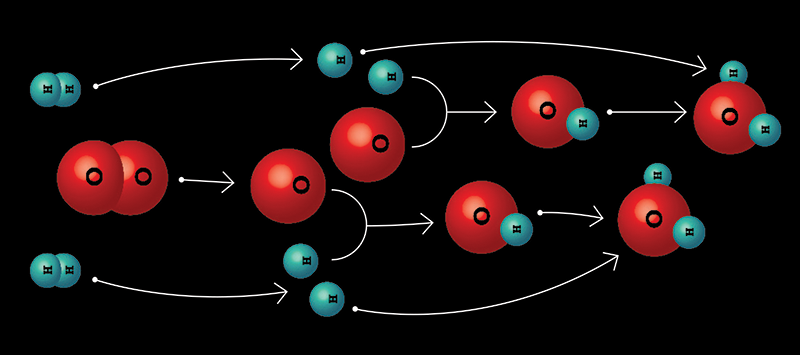
Experienced readers will say that the result of such an interaction will be water — and they are right if we talk about “normal” Earth conditions. In fact, the reaction of oxygen and hydrogen initially produces the hydroxyl radical OH, which can exist for years in rarefied clouds of interstellar gas. Probably, it must further react with one more hydrogen atom — and then we will get the H2O molecule we are used to. Of course, most of the water in space is in a gaseous state. But due to formation of so-called hydrogen bonds, its molecules can form complex structures, known under the common name of ice, even at a relatively high temperature (actually, at 00C, or at 273.15 Kelvin).
The structure of crystalline ice
However, most often it is not the crystalline ice that we are used to on Earth. It is believed that most of the ice in space is amorphous, that is, it does not have a clear structure. It is in this form, according to modern ideas, that it is included in the composition of comets that have existed in the space vacuum since the birth of the Solar System. Scientists hope to find this kind of ice in the cold traps at the poles of the Moon — in craters, whose bottoms are never touched by the rays of our Sun.
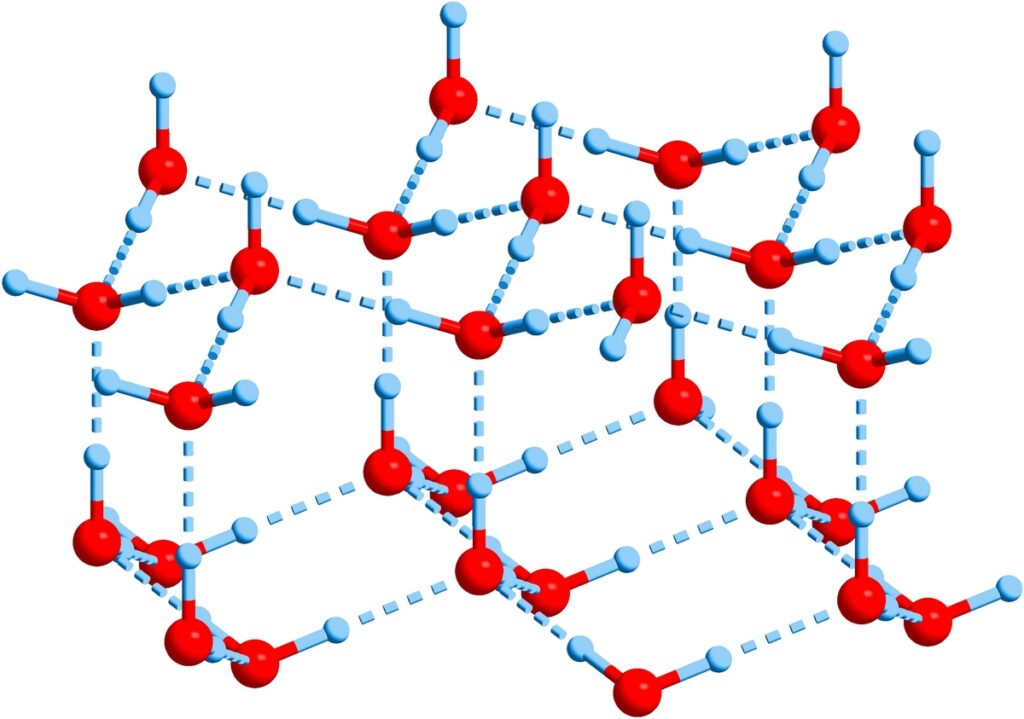
It would seem that in order for ice to stay on the surface of a celestial body, it is enough for it to have a temperature below 0°C (273.15 Kelvin). But it is not quite so. In a vacuum, even “at zero degrees”, ice evaporates quite quickly, and not only in a vacuum — remember how wet laundry dries in the cold: at first it hardens quite naturally, but later there is no trace of any freezing. That is why there is a concept of frost line in planetary science. In fact, this is an imaginary sphere, beyond which solar heat is no longer sufficient to effectively evaporate ice. According to various calculations, in our solar system its radius is from 2.7 to 3.1 AU. (400-460 million km).
An interesting example here can be the dwarf planet Ceres (1 Ceres), whose orbit lies near the frost line. In 2015, it was studied by the American Dawn automatic device. The equator of this celestial body is slightly inclined to the plane of the orbit, so in its equatorial regions there is no ice on the surface, but in the vicinity of the poles its signs can be traced very clearly.
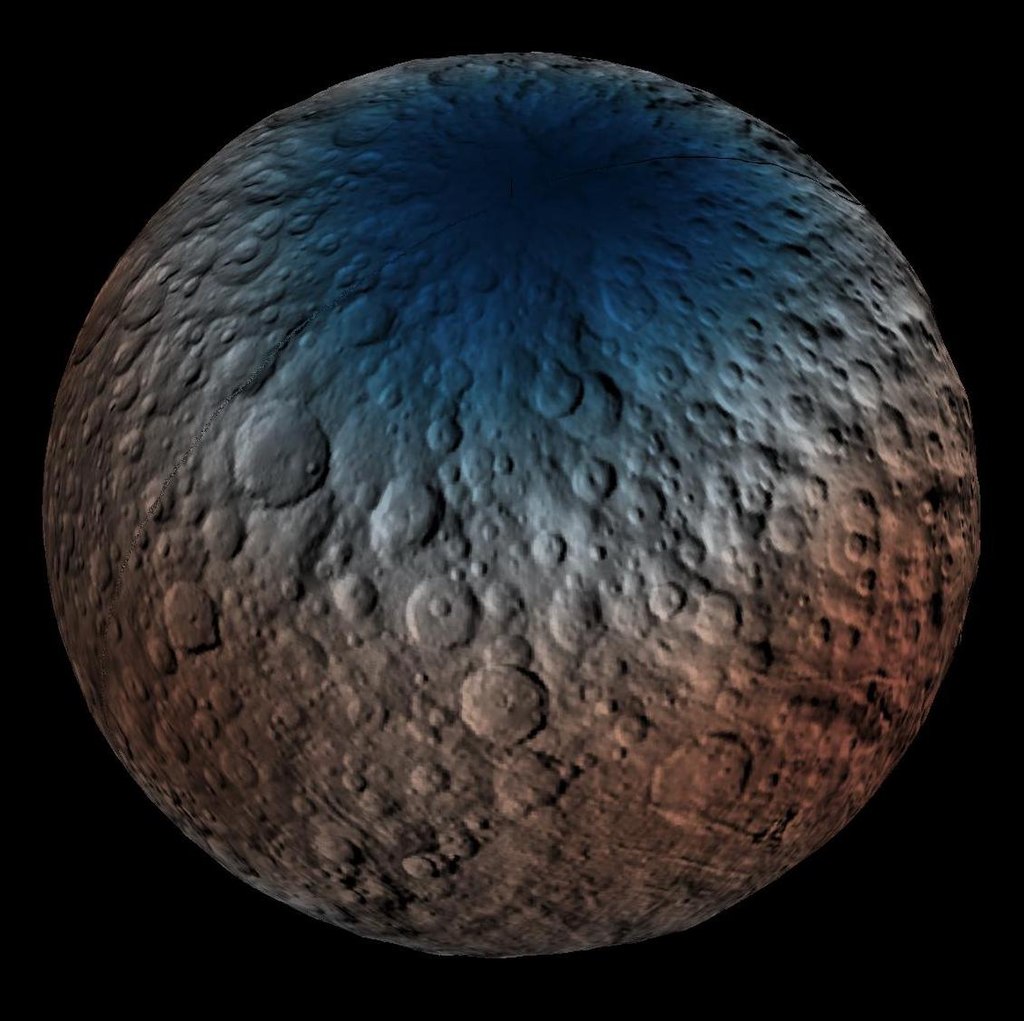
All the satellites of the planets of the Solar System, which are outside the frost line (with a few exceptions, the most famous of which is Io — the large moon closest to Jupiter), have an icy surface. Scientists tend to believe that similar bodies are quite often found in the vicinity of other stars. If they are more than 500 km in diameter, this means that they most likely passed the global ocean stage when they formed, so they are now covered not by amorphous ice, but by much stronger crystalline ice that we see here on Earth.
Unfortunately, it is quite difficult to prove the presence of water ice on the surface of a celestial body by remote methods, so astronomers are looking for indirect signs of its presence — for example, water in a gaseous state (it can be registered by spectral methods), which is the result of partial evaporation of glaciers. Research from a close distance using interplanetary vehicles can tell us much more. Europa, the smallest of the four Galilean moons of Jupiter, is the most spherical object of the Solar System: deviations of its shape from a regular sphere do not exceed a few hundred meters for a diameter of 3122 km. In fact, it is a huge “cosmic drop” with a stone core in its center whose surface is covered with a hundred kilometers thick ice crust. Gravimetric studies suggest that the Europa’s global sub-ice ocean contains at least twice as much salt water as all Earth’s seas and oceans combined.
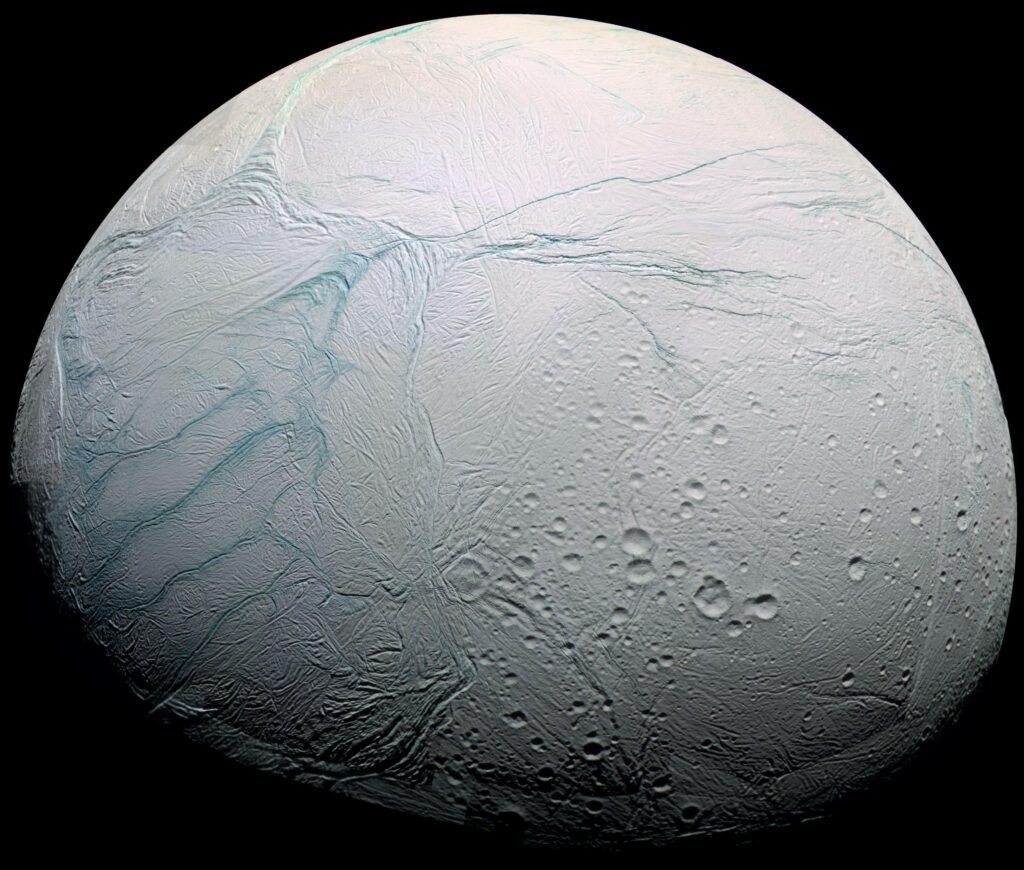
Bodies like Europa are considered extremely promising from the point of view of the search for life beyond our planet. The radioactive decay of heavy elements in their rocky cores, as well as tidal interactions (if they are natural satellites of other bodies or have their own massive moons) can provide them with enough energy to keep their cores in a liquid state for long periods of time. And this means that there will be conditions suitable for the emergence and evolution of living organisms, and they may even be similar to terrestrial ones (that is, composed of water solutions and carbon compounds), and therefore, it will be much easier for us to identify them. Therefore, it is Europa that will soon become the main goal of space missions searching for extraterrestrial life. In 2023, the European apparatus JUICE (JUPiter ICy moons Explorer) should be launched there. One of its scientific tasks, among other things, is the search for biomarkers — chemical compounds that can be products of the vital activity of microorganisms.
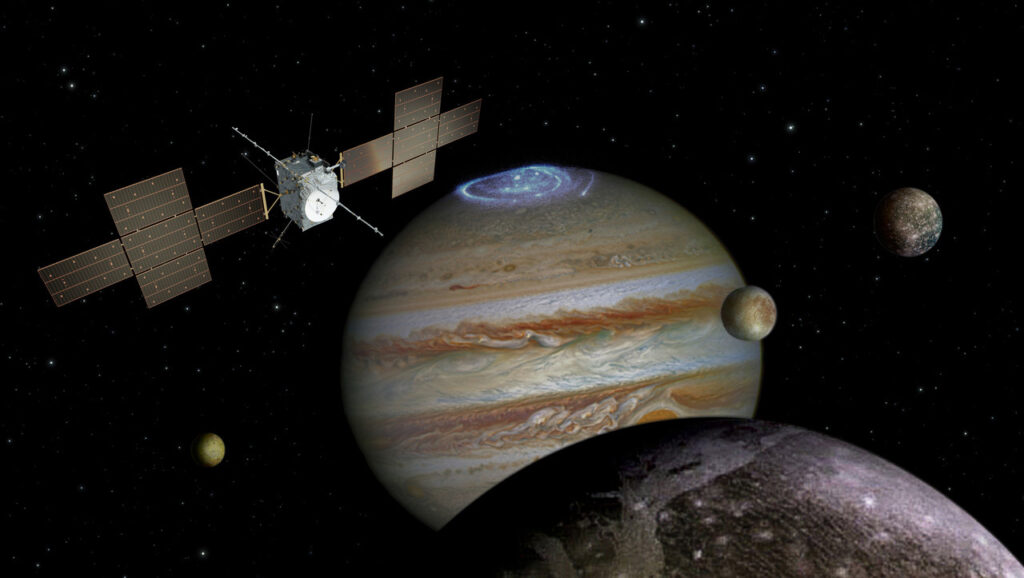
It is likely that such global ice-covered oceans may be present on many exoplanets orbiting outside the frost lines of their stars. As mentioned above, it is almost impossible to distinguish them from atmosphereless rocky bodies with the help of modern astronomical instruments. But their presence gives us hope that life in the universe can exist outside the so-called habitable zone — the region of space around a star, where the temperature conditions are favorable for the existence of liquid water on the surface. Actually, in order for liquid water to appear there, several additional conditions need to be fulfilled — the planet has to have a sufficiently dense atmosphere, and therefore a large mass (at least a quarter of the mass of the Earth)… It is not difficult to understand that exoplanets with all these characteristics will be found much less often than simple iced superdrops in independent orbits or as part of satellite systems of giant planets.