The history of astronomical discoveries is primarily the history of the invention and improvement of instruments for observing celestial bodies. The advent of optical telescopes has made it possible to see objects in more detail; the introduction of photographic plates has made it possible to detect much fainter stars and nebulae, as well as to “go beyond” the spectral range of human eye. Among the most important of these achievements, of course, is radio astronomy — a technique that once revealed to us a completely different universe with a huge variety of previously unknown phenomena and structures.
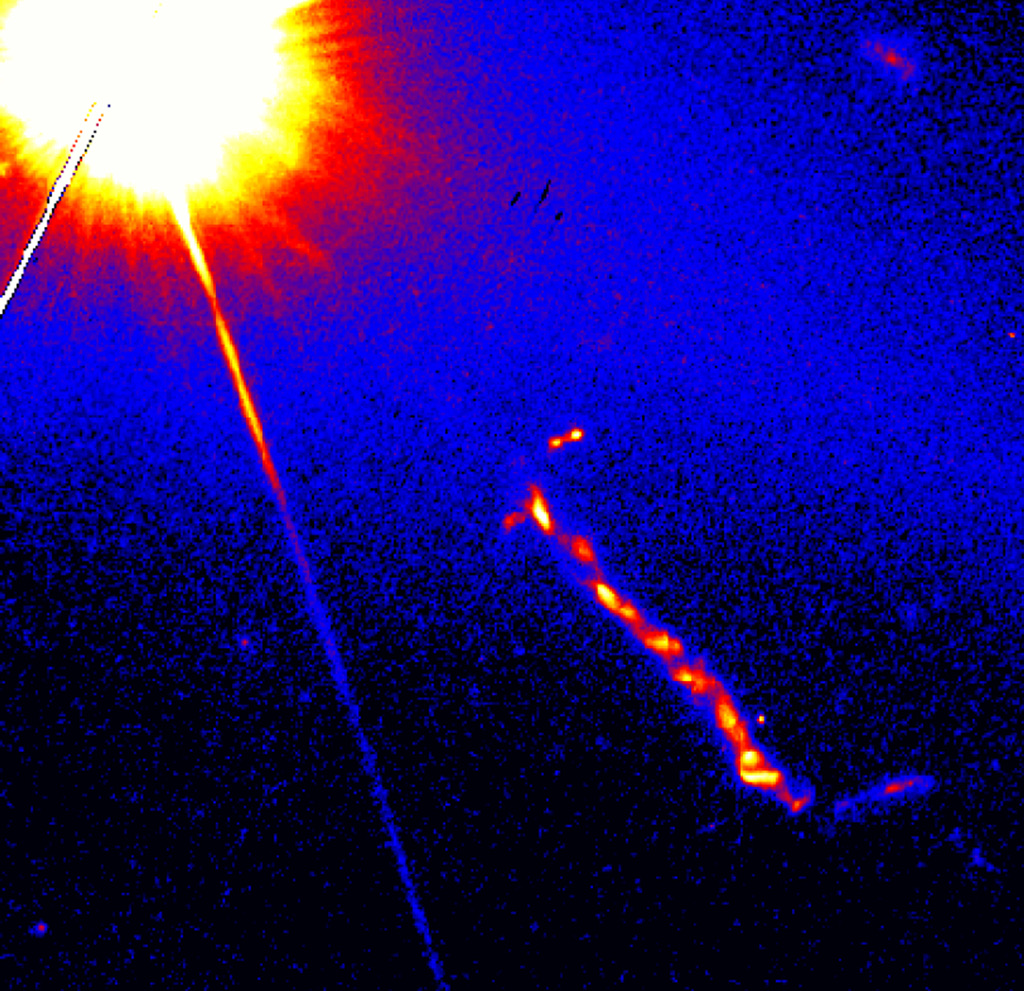
Even in the absence of the Moon and terrestrial light sources, the night sky is not really completely dark — it is constantly glowing due to radiation of atmospheric gases (but this glow is very weak and our eyes almost do not feel it). In the radio range, the entire celestial sphere also constantly emits at a frequency of about 160 GHz, which corresponds to a wavelength of 1.9 mm — this is the so-called cosmic microwave background (also known as “relic radiation”), a kind of “echo” of the Big Bang. Against this background, more “bright” clouds of interstellar and intergalactic gas stand out, as well as relatively compact areas of star formation. But much of the space radio waves come from point sources, the most mysterious objects, many of which have yet to reveal all their secrets. These include quasars. In fact, their very name, which is an abbreviation of quasi-stellar radio source, contains a hint of “star” appearance.
The fact is that the first radio telescopes had a very low resolution — they allowed to determine very roughly the direction from which the radiation comes and the angular size of its source. When these data improved sufficiently, astronomers realized that some of the radio sources were very small. Gradually, they began to be identified with objects found during observations in other spectral ranges. Often these turned out to but young stars that were just forming, or vice versa — the remnants of supernovae, which are formed after active life of massive luminaries comes to an end. But in some cases, literally nothing could be found at the site of the radio source.
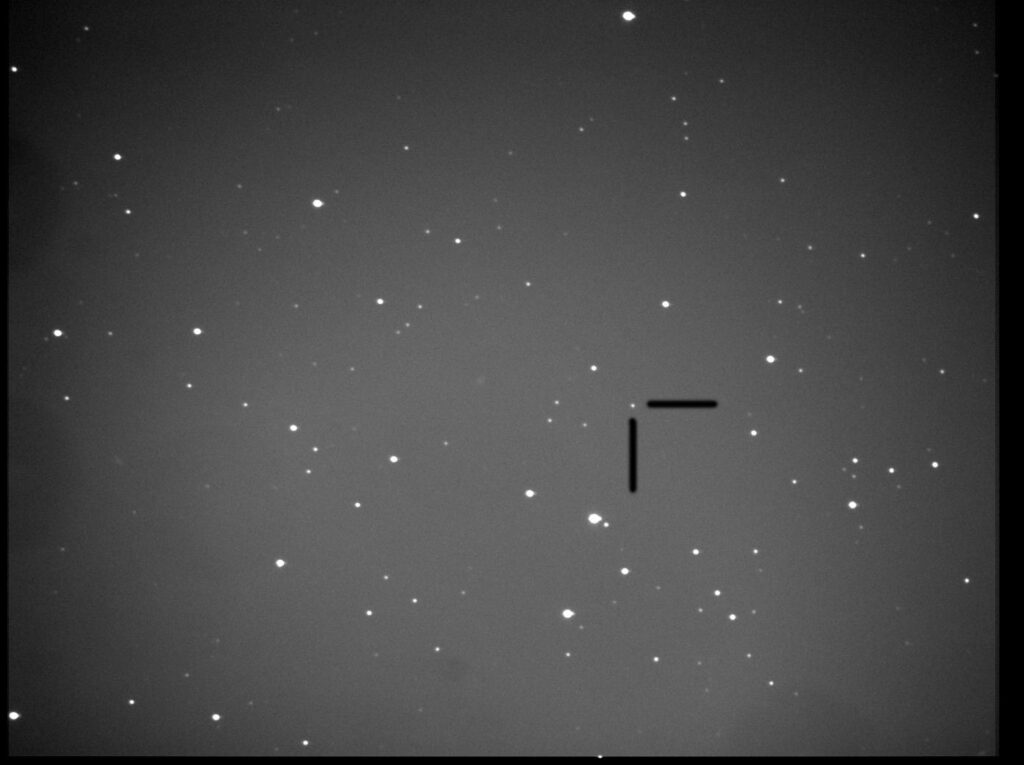
In the middle of the last century, all the then-known “celestial radio transmitters” were consolidated in the so-called Third Cambridge Catalogue and began to be marked with a number with the prefix “3C”. In 1960, using a new technique of interferometry, astronomers Alan Sandage and Thomas Matthews managed to accurately determine the position of the source 3C 48, discovered shortly before. It was matched with a faint star in the constellation Triangulum, and it was 16 magnitudes — i.e. 10,000 times weaker than objects visible to the naked eye — but nevertheless it sent a powerful stream of radio waves into space.
An even more interesting story happened with source 3C 273. It is located in the constellation of Virgo, somewhere near the ecliptic, so astronomers have suggested that it may occasionally be obscured by the moon as it orbits. Such “screenings” were indeed observed. The difference between the moments of disappearance and subsequent reappearance of the radio signal, measured from two different points on the earth’s surface, allowed us to calculate the exact coordinates of 3C 273 and find its optical equivalent, which this time had a high enough brightness — 12.6 m. And this allowed obtaining images of its spectrum through contemporary technology. This is where the main surprise happened: this spectrum turned out to be unlike any seen before.
Even before the heyday of radio astronomy, scientists have already got convinced due to the work of Edwin Hubble, that our Milky Way is just one of a huge number of stellar systems inhabiting the ever-expanding universe, and on a fairly large spatial scale the speed of mutual moving away of two objects in it is proportional to the distance between them. The speed of “flying away” can be measured by spectral methods — by shifting the characteristic lines of radiation of individual chemical elements to the longer wavelength end of the spectrum (it is also called “redshift”, and denoted by the Latin letter z). In the spectrum of 3C 273, this shift was so large that the lines of hydrogen radiation, which are usually in the ultraviolet range, “crawled” to its visible part. For 3C 48, this figure was even higher and corresponded to a distance of almost 4 billion light years. Astronomers have never encountered such distant objects…

In order to emit a visible glow at such a distance (even greater glow than that of many stars in our Galaxy), the radiation source must be incredibly powerful. For example, the already mentioned quasar 3C 273 is four trillion times brighter than our sun. It was impossible to imagine that so much energy was emitted by an “ordinary” star (even a very massive and hot one). We had to look for more efficient mechanisms of energy release, including, for example, the presence in some parts of the universe of local accumulations of antimatter, which constantly annihilates with “normal” matter with complete conversion of mass into energy. Finally, scientists found the best explanation: it was the fall of large amounts of matter on supermassive black holes. This mechanism was proposed in 1964 by the American astrophysicist of Austrian descent Edwin Salpeter and the Soviet physicist Jacob Zeldovich.
With the advent of a large number of powerful optical and radio telescopes, the number of known radio sources with large values of z — this is how we can describe the main characteristics of objects called “quasars” — began to grow rapidly, and this, in turn, necessitated some differentiation within of this class. For example, a special category includes a source that was originally considered a variable star and was designated BL Lacerta. When a few more similar objects were found, they were called “blazars”. At the same time, the technique of observations was improved. In 1982, two “petals” in the quasar 3C 48 were first seen in an image synthesized by several radio antennas. We now know that these are so-called jets, high-velocity flows of matter that fall into a supermassive black hole and then is thrown back into space as a result of interaction with the surrounding accretion disk. Similar structures (but smaller in size) were already observed at that time in other, closer galaxies, containing in their central regions black holes with masses of millions of suns. This has become one of the main evidences of the “black-hole ” mechanism of quasar radiation.
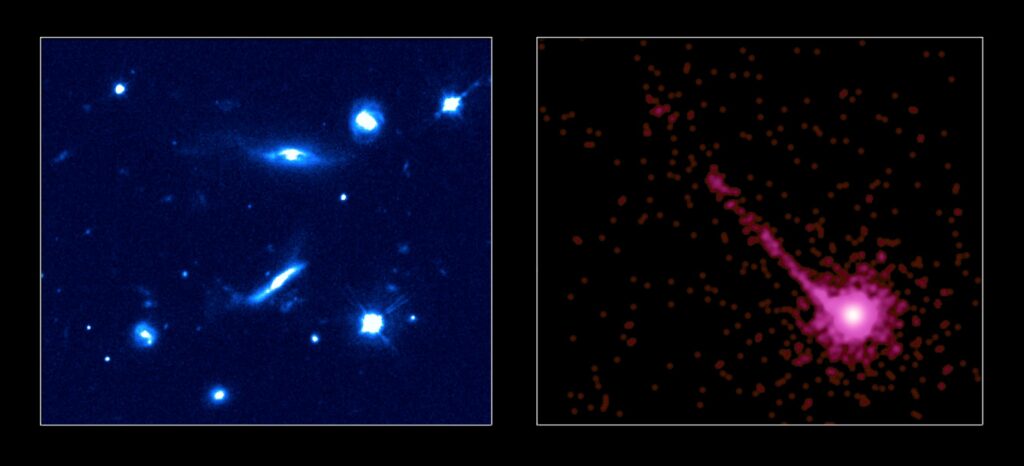
In 1990, the Hubble Space Telescope began its work, and soon took another step toward understanding the nature of these mysterious objects by photographing a small, irregularly shaped nebula surrounding the bright “core” of the 3C 273 quasar. It became evident that it is the nucleus of a very distant galaxy that is in the process of formation.
Later, more and more quasars were discovered, and the redshift in the spectra of some of them indicated that they were located at great distances from us. The current “record holder” — ULAS J1342 + 0928 2017 in the constellation of Boötes — has z = 7.54, ie the light from it went to us more than 13 billion years, and we observe it at a time when only 690 million years has passed since the birth of our universe. Of course, all this time the quasar did not “stand still”, but continued to move away, so now the distance to it is almost 30 billion light years.
The more sensitive our astronomical instruments became, the more quasars were found. It became clear that this is actually quite a typical phenomenon for the early stages of the evolution of the universe. It is very likely that all large galaxies (including our Milky Way) underwent a “quasi-like” phase during their formation, when their central supermassive black hole absorbed vast amounts of surrounding matter and grew rapidly, forming the main “attraction” point of the future stellar system.
The study of quasars was accompanied by other interesting discoveries. For example, near some of them there were “twins” with a completely similar spectrum and brightness curve, “shifted” to the past for a fixed period of time. Between such “quasar twins” there is a closer massive galaxy. Scientists have realized that in this case we are dealing with the effect of gravitational lensing — the distortion of light rays by gravity of a massive object between the radiation source and the observer. As a result, photons emitted by the quasar travel to us in two routs of slightly different lengths, forming two almost identical images. However, there may be more such images: in 1985, a group of researchers led by John Huchra photographed a distant star system in the Pegasus constellation, surrounded by four “reflections” of the quasar on which it was accidentally projected. Because this effect was predicted by Einstein’s Theory of Relativity, this category of objects (which now has become a classic case) is called Einstein’s Cross.

Gravitational lensing makes it possible to learn more about the properties of quasars, to define more accurately the distance to them, and to discern weaker objects that, in the absence of a “natural lens,” would be beyond the reach of available astronomical instruments. Many telescopes, both terrestrial and space, are currently involved in the study of these mysterious radio sources (the space telescopes allow observations in the ultraviolet, infrared, and X-ray ranges of the spectrum, for which the Earth’s atmosphere is not transparent). The total number of known quasars has already exceeded 200,000 and continues to grow. They conceal many more mysteries, while providing answers to other questions related to the evolution of our universe and its structure on a large scale. For example, with their help, scientists are now trying to find out when the so-called reionization of intergalactic neutral gas was completed — this information is very important when modeling the mechanisms of formation of the first stars, planetary systems and galaxies. Specialized observatories for recording gravitational waves (the first of which were the American LIGO and European Virgo) are an additional powerful tool here, which can “see” the effects of interaction and collision of supermassive black holes. Astronomers have only just begun to master this technique, but it is clear that it is also able to give us many discoveries.
Follow us on Twitter to get the most interesting space news in time:
https://twitter.com/ust_magazine